Photobiology and photophysics of biomolecules
The vital activity of living systems under the conditions of solar radiation causes great interest in the photonics of biological molecules. In the course of evolution, nature has created unique molecular systems that effectively interact with solar radiation, such as, for example, the retina of the eyes of animals, the light-harvesting antenna of photosynthetic reaction centers of plants and bacteria, in which light-absorbing pigments are functional molecules. Understanding the physics of quantum processes of interaction of light with biomolecules and medicinal substances is extremely important not only from an academic, but also from a practical point of view, both for modern biomedicine and for the rapidly developing branch of bioinspired materials science.
Photodynamic therapy is based on the destruction of tumor cells with highly reactive substances (for example, singlet oxygen ) generated in tissues by photosensitizers (Fig. 1).
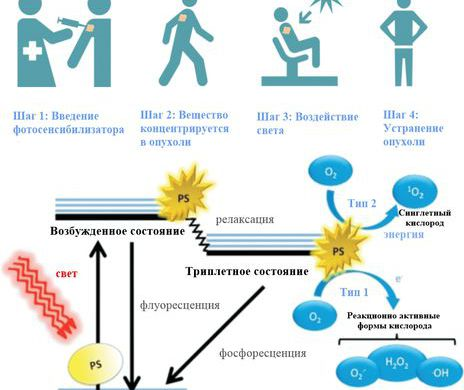
Fig. 1. The principle of photodynamic therapy (source).
Photochemistry and photophysics of sensitizers (compounds that generate singlet oxygen (1О2) and are used in photodynamic therapy (PDT) of cancer) have been studied in sufficient detail and are currently being studied in many laboratories around the world. Probably, in the coming years, photosensitizers will become even more in demand: due to the growth of bacterial resistance to antibiotics, sensitizers will be widely used in antimicrobial PDT [Wainwright et al, 2017].
The quantitative structure-property relationship (QSPR) method is used in photochemistry to predict the wavelength of the absorption maximum [Chaudret et al., 2015], fluorescence intensity [Riahi et al., 2009], photoinduced toxicity, constants the rate of photolysis and the quantum yield of photolysis [Zhang, 2013]. Until now, no attempts have been made to use QSPR to predict the quantum efficiency (QE) of 1О2 generation. In this regard, our research using methods of computational chemistry, QSPR and machine learning in photochemistry can be considered pioneering, since no one has done this before. In silico models make it possible to predict whether the developed compound will have a high CV and whether it is worth spending time and resources on its synthesis. In this regard, using QSPR, we investigated the generation of 1О2 by pterins and flavins [Buglak et al, 2016], psoralens and angelicins [Buglak, Kononov, 2018], porphyrins and metalloporphyrins [Buglak et al, 2020], boron dipyrromethanes (BODIPY) [Buglak et al, 2021] (Fig. 2).
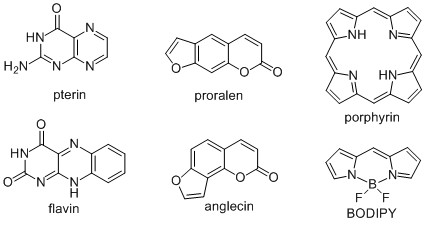
Fig. 2. Chemical formulas of the main investigated compounds.
QSPR analysis was performed using various machine learning methods: multiple linear regression (MLR), support vector machine (SVM), and random forest (RF). We have shown that QSPR is useful for predicting the activity of potentially photoactive drug compounds with clinical significance (Fig. 3). Overall, we expect that the approach we are developing should begin to be useful and applied in photomedicine in the coming years.
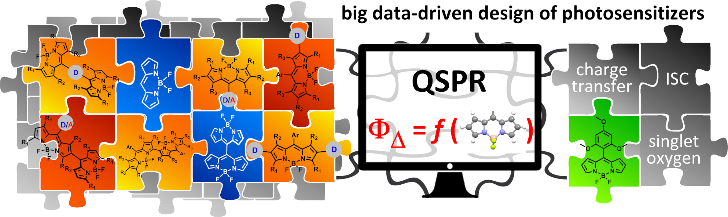
Fig. 3. Using Big Data to design BODIPY photosensitizers (source).
Experimentally and theoretically, we plan to research the development of new sensitizers based on metal clusters and carbon quantum dots .
In addition, other works on the study of the photochemistry of biomolecules are being carried out in the laboratory. For example, the photophysical properties of an important biological coenzyme, tetrahydrobiopterin (H4Bip), have been investigated by the methods of quantum chemistry. In recent years, it has been shown that H4Bip can play the role of a photoreceptor molecule in plants and cyanobacteria. Our calculations have demonstrated the possibility of ultrafast internal conversion of the energy of photoexcitation Н4Бip. The possibility of the participation of H4Bip as an electron donor in photoinduced electron transfer (PET) was also assessed [Buglak, Telegina, 2018]. Using density functional theory (DFT), the possibility of redox photoreactions between pterins and molecular oxygen was assessed [Buglak et al, 2019],which is important from the point of view of the use of these compounds in PDT.
- Wainwright M, Maisch T, Nonell S, Plaetzer K, Almeida A, Tegos GP, Hamblin MR. Photoantimicrobials-are we afraid of the light? Lancet Infect Dis. 2017; 17: e49-e55.
- Chaudret R, Kiss CsF, Subramanian L. Prediction of absorption wavelengths using a combination of semi-empirical quantum mechanics simulations and quantitative structure – property relationship modeling approaches. J Photochem Photobiol A. 2015; 299: 183-188.
- Riahi S, Beheshti A, Ganjali MR, Norouzi P. Quantum chemical calculations to reveal the relationship between the chemical structure and the fluorescence characteristics of phenylquinolinylethynes and phenylisoquinolinylethynes derivatives, and to predict their relative fluorescence intensity. Spectrochim Acta A. 2009: 74 (5): 1077-1083.
- Buglak AA, Telegina TA, Kritsky MS. A quantitative structure-property relationship (QSPR) study of singlet oxygen generation by pteridines. Photochem Photobiol Sci. 2016; 15: 801-811.
- Buglak AA, Kononov AI. Triplet state generation by furocoumarins revisited: a combined QSPR/DFT approach. New J Chem. 2018; 42: 14424-14432.
- Buglak AA, Filatov MA, Hussain MA, Sugimoto M. Singlet Oxygen Generation by Porphyrins and Metalloporphyrins Revisited: a Quantitative Structure-Property Relationship (QSPR) Study. J Photochem Photobiol A. 2020; 403: 112833.
- Buglak AA, Charisiadis A, Sheehan A, Kingsbury CJ, Senge MO, Filatov MA. Quantitative Structure – Property Relationship Modeling for the Prediction of Singlet Oxygen Generation by Heavy-atom-free BODIPY Photosensitizers. Chem Eur J. 2021.
- Buglak AA, Telegina TA. A Theoretical Study of 5,6,7,8-Tetrahydro-6-Hydroxymethylpterin: Insight into Intrinsic Photoreceptor Properties of 6-Substituted Tetrahydropterins. Photochem Photobiol Sci. 2019; 18: 516-523.
- Buglak AA, Telegina TA, Vorotelyak EA, Kononov AI. Theoretical study of photoreactions between oxidized pterins and molecular oxygen. J Photochem Photobiol A. 2019; 372: 254-259.